In many countries, coal is the primary source of energy. Although the safety situation in coal mines around the world has substantially improved over the years, serious accidents in coal mines still occur regularly, and the coal mine safety case remains critical.
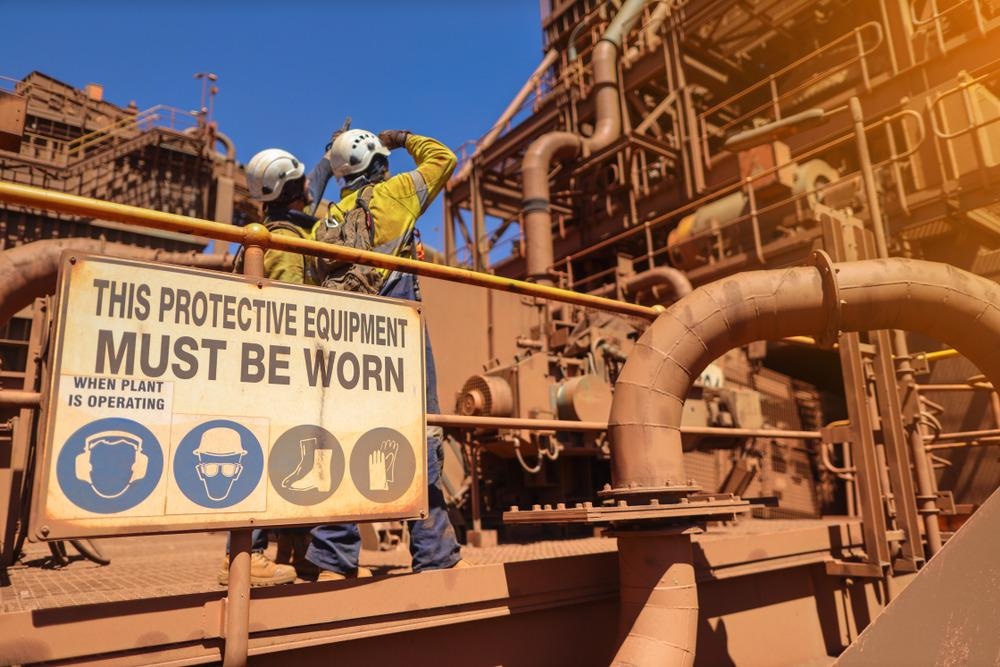
Image Credit: King Ropes Access/Shutterstock.com
In China, 55 significant coal mine thermal accidents occurred between 2010 and 2021, resulting in 1074 fatalities, including 27 incidents (49.09%) in the gob and working face, resulting in 508 fatalities (47.3%).
Due to the obvious geological complexity of underground mining, avoiding gas explosions in coal mines has long been a priority and a challenge.
To effectively prevent gas explosions in the coal mining business, more research into the ignition sources in gobs is required.
After mining, a gob is a unique place formed by the collapse and accumulation of rock on the surface of a coal seam. The level of filling is determined by a variety of criteria, such as the height of the rockfall down (seam thickness or mining height), the kind of roof rock, and rock strength features, as well as the distance from the front coal working surface and the depth of mining exploitation.
Previous scholars have looked at the electrodynamic effects of rocks in precise detail. These investigations, however, have mostly targeted the luminescence of rocks in the air and have not taken into account the methane atmosphere.
Electrical ignition properties generated by load crack discharge during coal roof mining are poorly known.
As a result, a paper, published in ACS Omega, investigates the piezoelectric effect of roof deformation and fracture sparking the methane qualities to provide accurate ideas for the prevention and management of gas explosions in the gob. This will be critical for disclosing the process of gas explosions in the stope.
Methodology
The rock samples were taken from the Renlou coal mine’s roof. To eliminate moisture from the pores, the rock samples were put in a drying cabinet at 80 °C for 12 hours.
Figure 1 depicts the experimental setup. The uniaxial compression experiments were carried out on a hydraulic universal testing machine with loading rates of 0.5 and 5 kN/s.
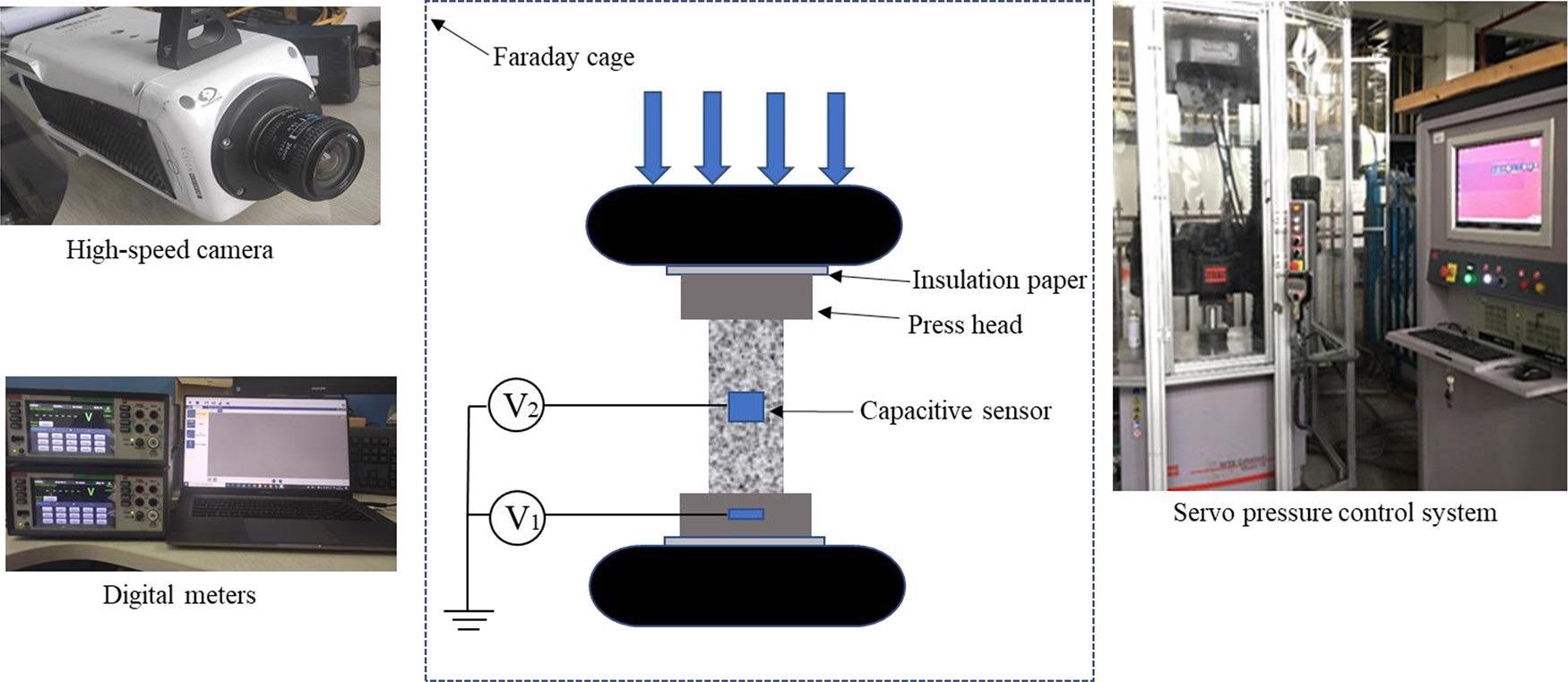
Figure 1. Schematic diagram of the rock sample discharge experiment system. Image Credit: Wang, et al., 2022
Results and Discussion
As shown in Figure 2, the quartz source of the Renlou mine sandstone is elevated, accounting for 63.7%, with feldspar (Na2O-Al2O3-6SiO2) source accounting for 28.2%, generating the standard skeletal structure of the sandstone.
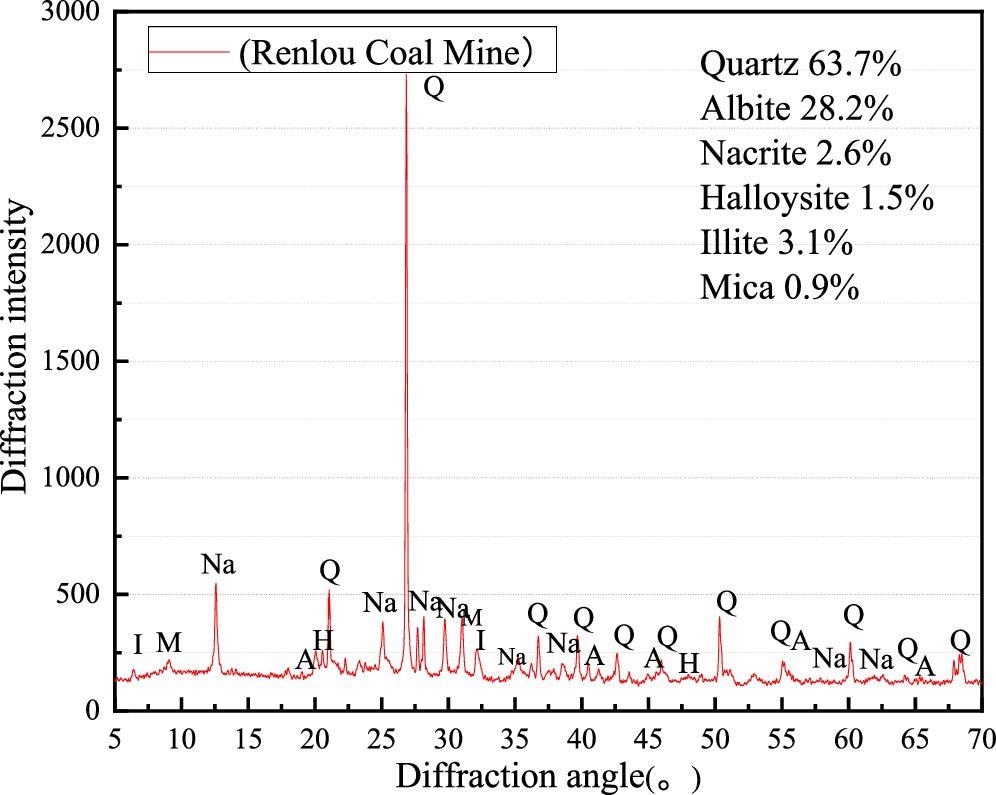
Figure 2. XRD results of roof sandstone in the Renlou coal mine. Image Credit: Wang, et al., 2022
Figure 3 shows the SEM findings of the rock sample at various magnifications. On the surface of the quartz sample, there are numerous uneven, columnar, or granular bright white spots. It possesses sedimentary rock properties, such as fine grains and small angles, and the grains are tightly bonded to the cementation, resulting in high compressive strength.
This well-developed lamellar structure implies that these rocks are substantially anisotropic and capable of producing piezoelectric effects at both the microscopic and macroscopic levels.
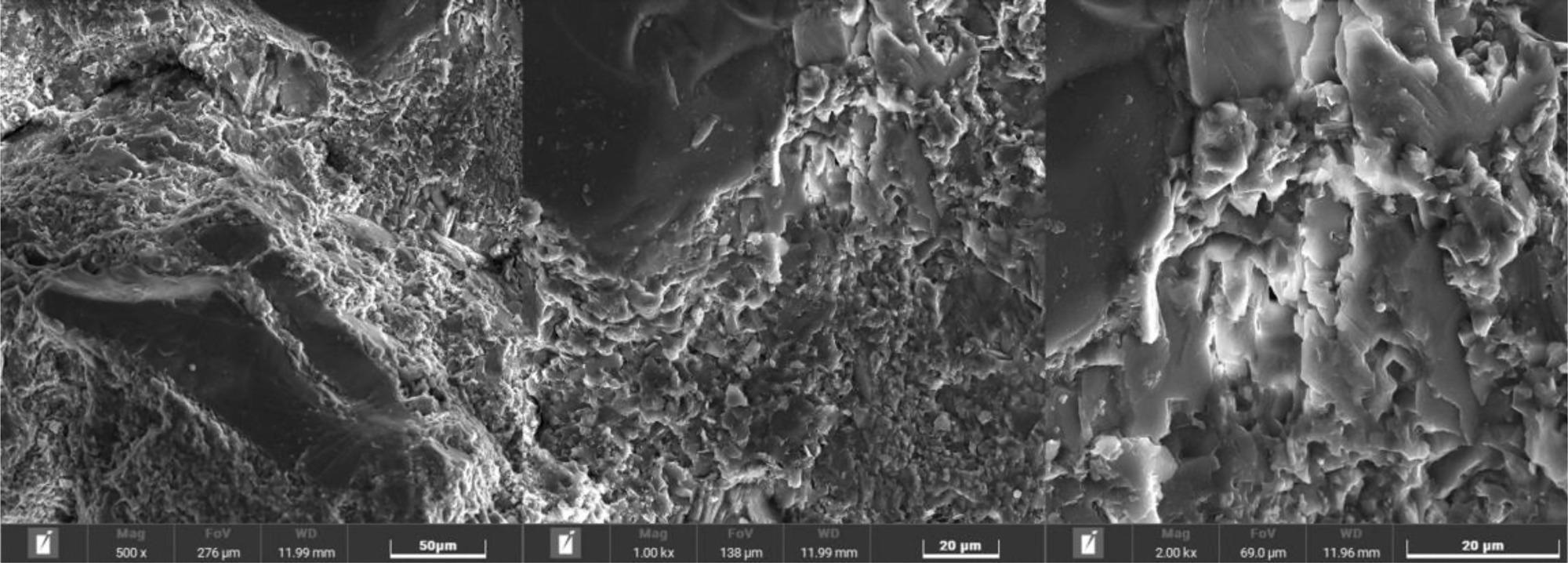
Figure 3. SEM images of the rock samples at different magnifications. Image Credit: Wang, et al., 2022
Figure 4 depicts the discharge patterns of Renlou mine sandstones at 0.5 and 5 kN/s loading rates. Table 1 presents the maximum voltage, loading duration, and peak pressure of the Renlou mine sandstone during the destruction phase at various loading rates.
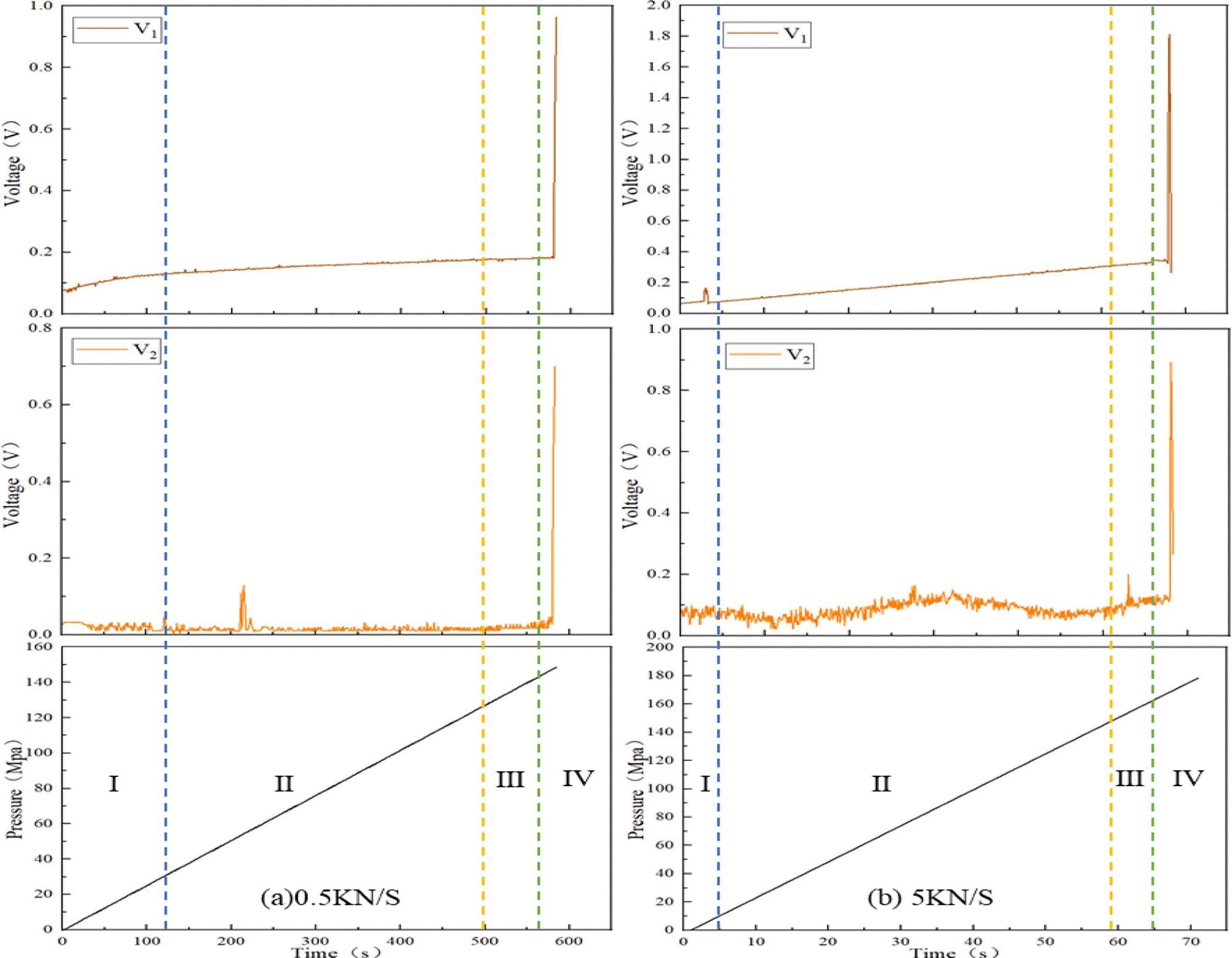
Figure 4. Discharge patterns of Renlou mine sandstones at two loading rates of 0.5 and 5 kN/s. Image Credit: Wang, et al., 2022
Table 1. Peak Voltage, Loading Time, and Peak Pressure during the Damage Phase of the Renlou Mine Sandstone at Different Loading Rates. Source: Wang, et al., 2022
Loading rates
(kN/s) |
Specimen |
V1max
(V) |
V2max
(V) |
Tmax
(s) |
Pmax
(MPa) |
0.5 |
1 |
1.1 |
0.52 |
582.5 |
148.4 |
2 |
1.3 |
0.7 |
610.3 |
155.4 |
3 |
1.21 |
0.59 |
570.2 |
145.2 |
5 |
1 |
1.78 |
0.826 |
70.3 |
178 |
2 |
1.91 |
0.891 |
74.1 |
187.7 |
3 |
1.83 |
0.89 |
75 |
189.9 |
The polarization distribution of charges during the first 3 phases of compression and distortion of the rock can be simplified to a parallel plate capacitor, as illustrated in Figure 5, if the entire rock is treated as a whole during the process of compression.
The faster the rate of pressure exerted, the quicker the electric field varies, the stronger the magnetic field produced, the stronger the energy collected, and the higher the number of charges discharged at the point of fracture, as shown in Figure 4a, b.
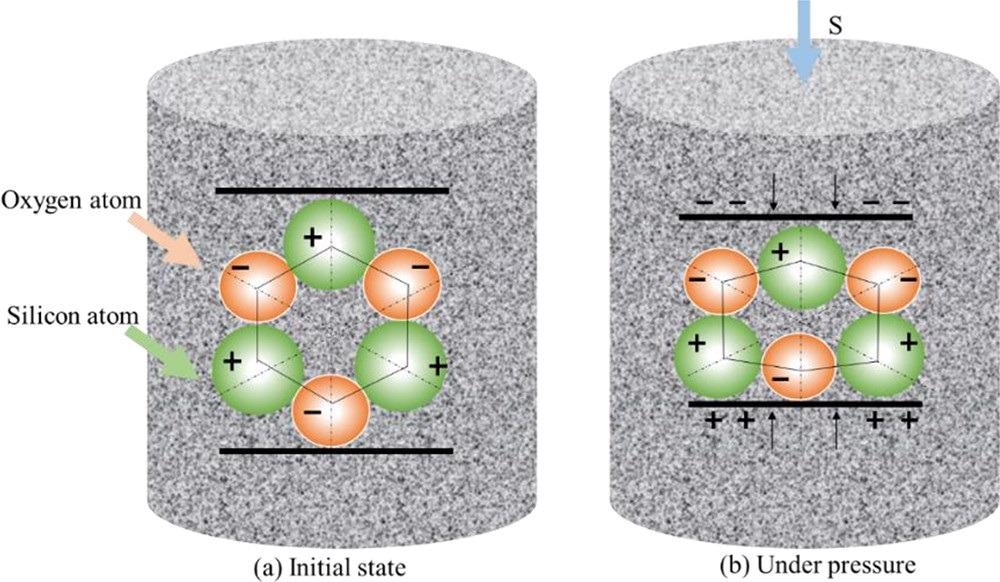
Figure 5. Polarization distribution of charges. Image Credit: Wang, et al., 2022
Figure 6 depicts the typical spurting sparks produced by the sandstone as a result of the electron avalanche event.
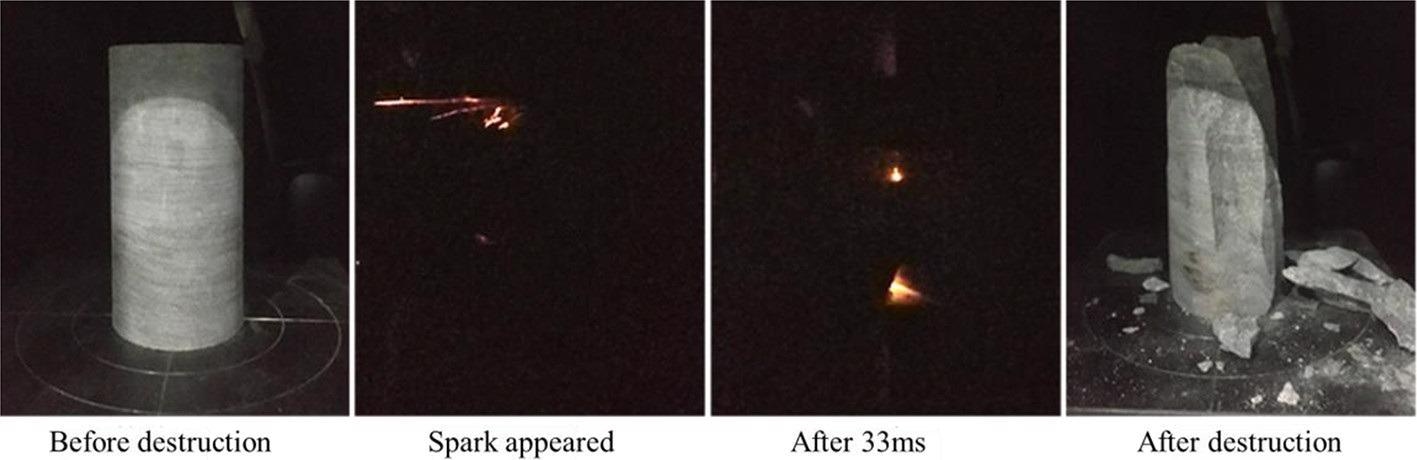
Figure 6. Spark produced by the roof sandstone. Image Credit: Wang, et al., 2022
Figure 7 depicts the destruction of positive and negative electrons into photons.
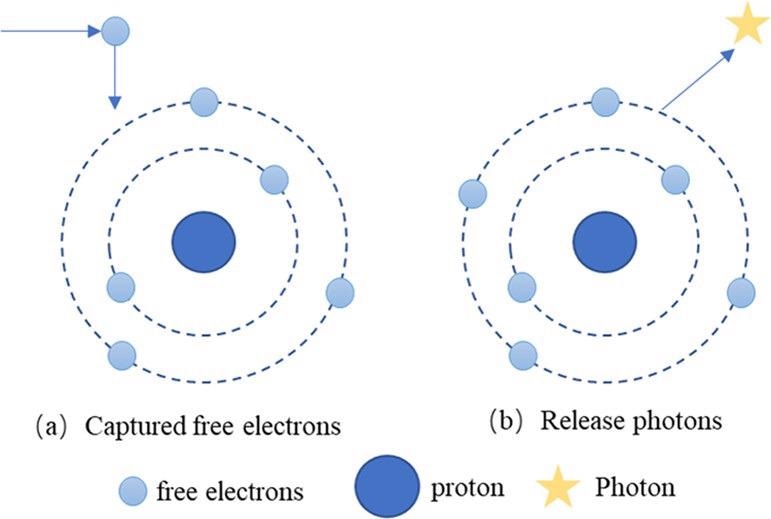
Figure 7. Photon emission process. Image Credit: Wang, et al., 2022
The thermal concept of ignition and the electrical concept of ignition are two widely accepted hypotheses for the process of electrical spark ignition. The gas mixture burns as a result of the chain reaction.
In conclusion, the researchers note that in the process of gas igniting by the piezoelectric effect of rock, two mechanisms operate simultaneously.
Figure 8 depicts the time scales of particle reactions.
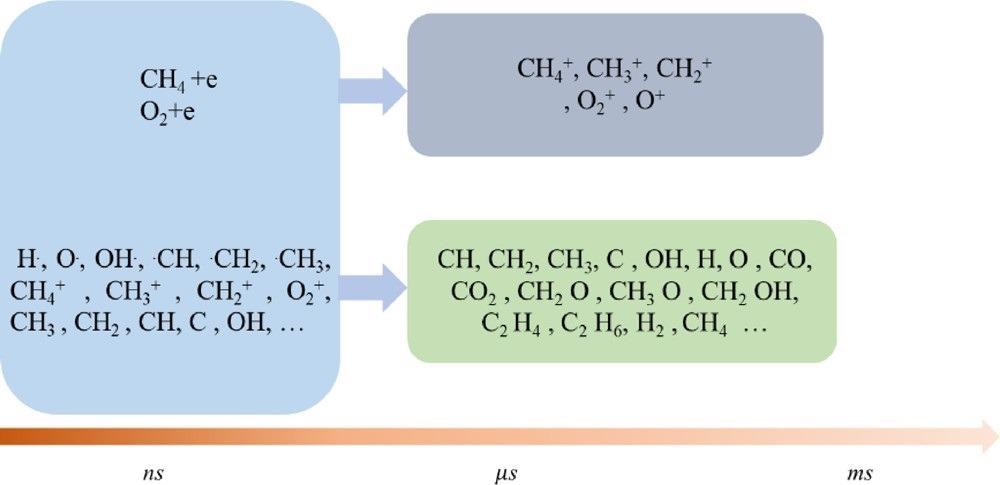
Figure 8. Time scale of particle reactions. Image Credit: Wang, et al., 2022
Three persons were killed in a methane explosion in the Renlou coal mine in 2014. The methane explosion happened unexpectedly. Following the disaster, mine technicians tried several steps to prevent the gob’s coal from self-igniting, but four gas explosions occurred in quick succession.
Figure 9 depicts the accident site of the gas explosion. Table 2 shows the actual explosion times.
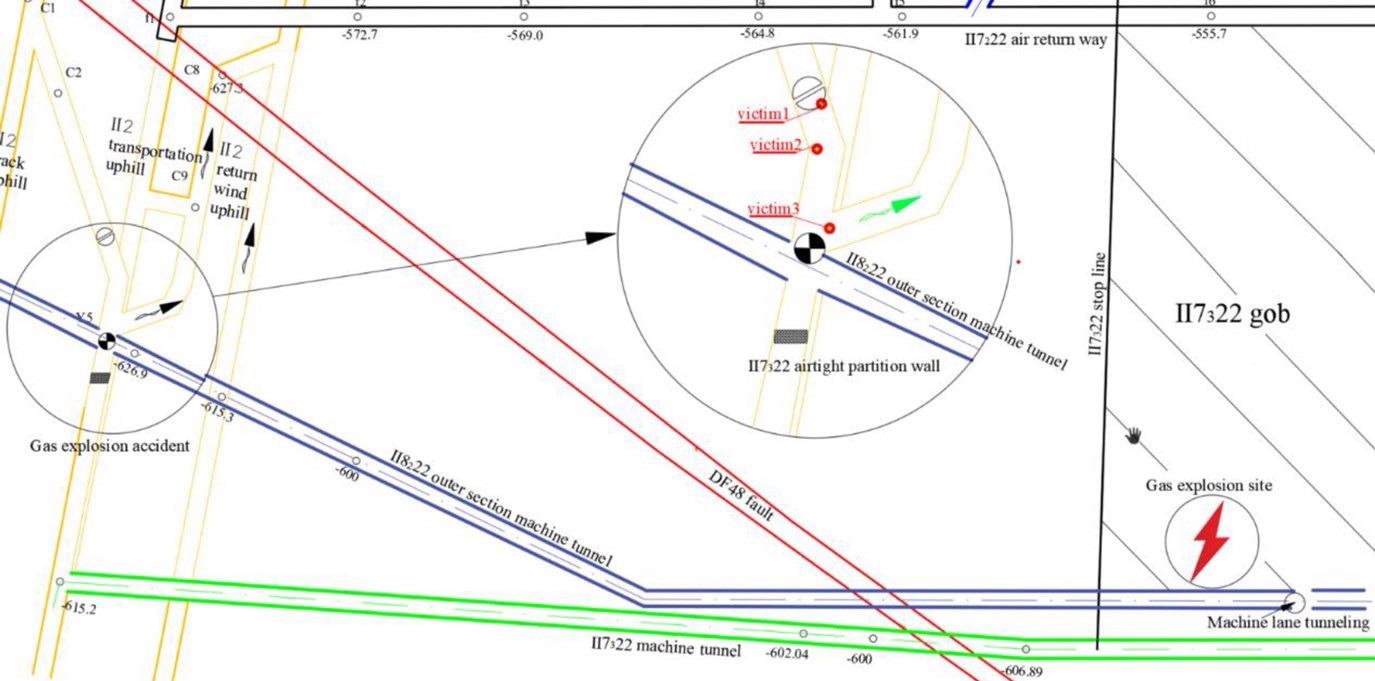
Figure 9. Gas explosion accident area. Image Credit: Wang, et al., 2022
Table 2. Exact Explosion Times of the II7322 Gob. Source: Wang, et al., 2022
Explosion |
Date |
Time |
1st explosion |
12 March |
15:09 |
2nd explosion |
15 March |
18:28 |
3rd explosion |
15 March |
20:18 |
4th explosion |
15 March |
22:09 |
5th explosion |
17 March |
17:11 |
There was no definite determination concerning the ignition source after the disaster. Some analysts assume the gas-air mixture was ignited by the leftover coal in the II7322 gob self-igniting.
Figure10a depicts the trend of data collected by the CO concentration sensor at the II7322 air return way’s airtight partition wall.
Figure 10b illustrates the CO sensor data variation trend in the II2 mining area’s major return airway. Figure 10c exhibits the CO sensor data variation trend in the II8222 air return way’s outer part.
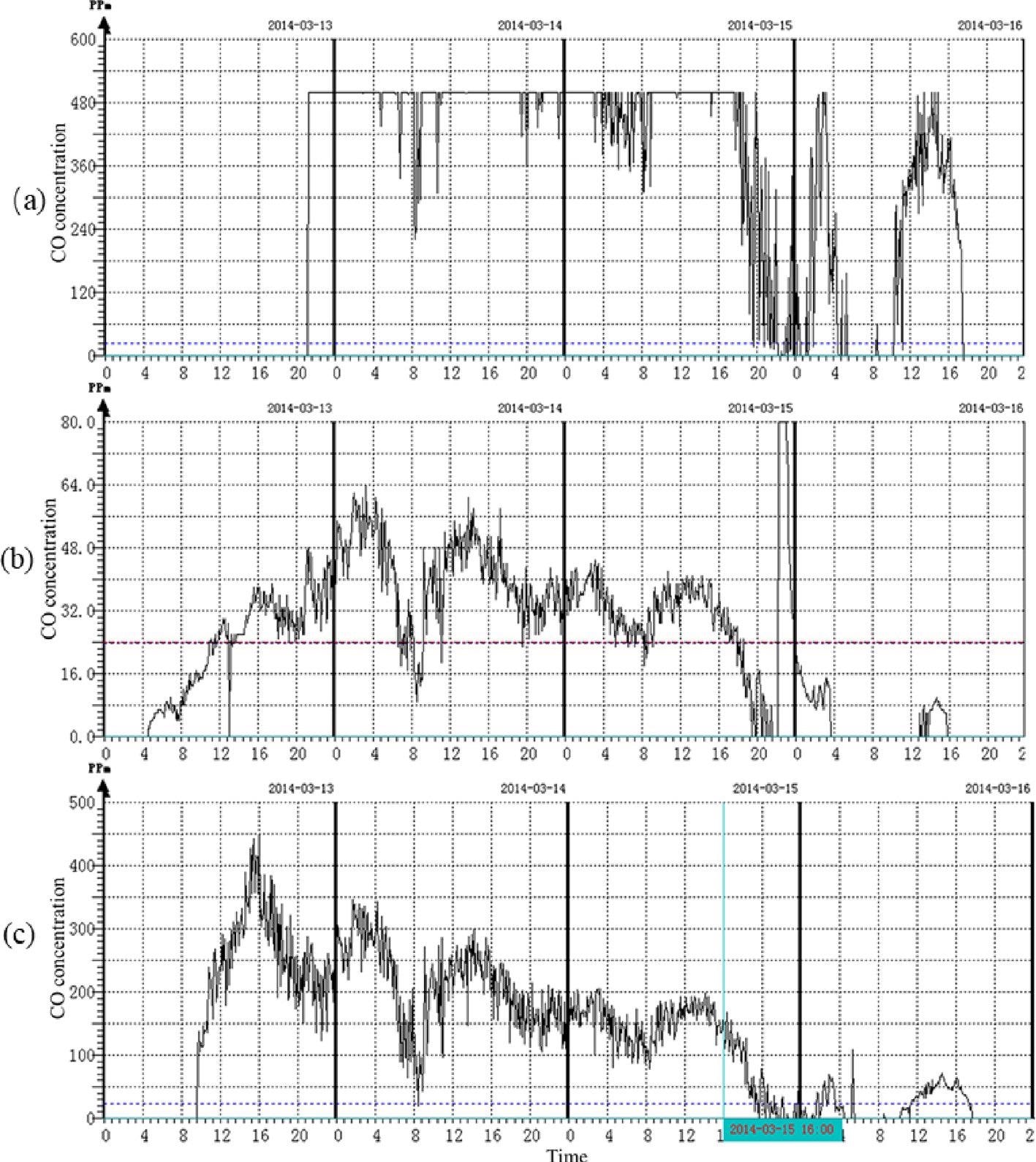
Figure 10. Variation trend of CO sensor data. (a) Airtight partition wall of the II7322 air return way; (b) main return airway of the II2 mining area.; (c) outer section of the II8222 air return way. Image Credit: Wang, et al., 2022
Three individuals were killed by the shock wave while transferring material for the II8222 machine tunnel’s outer section outside the airtight partition wall, whereas the II7322 air return way’s airtight partition walls were uninjured, as illustrated in Figure 9.
The DF48 fault is intersected by the II7222 machine tunnel, II7322 machine tunnel, and II8222 outer section machine tunnel, as depicted in Figure 11.
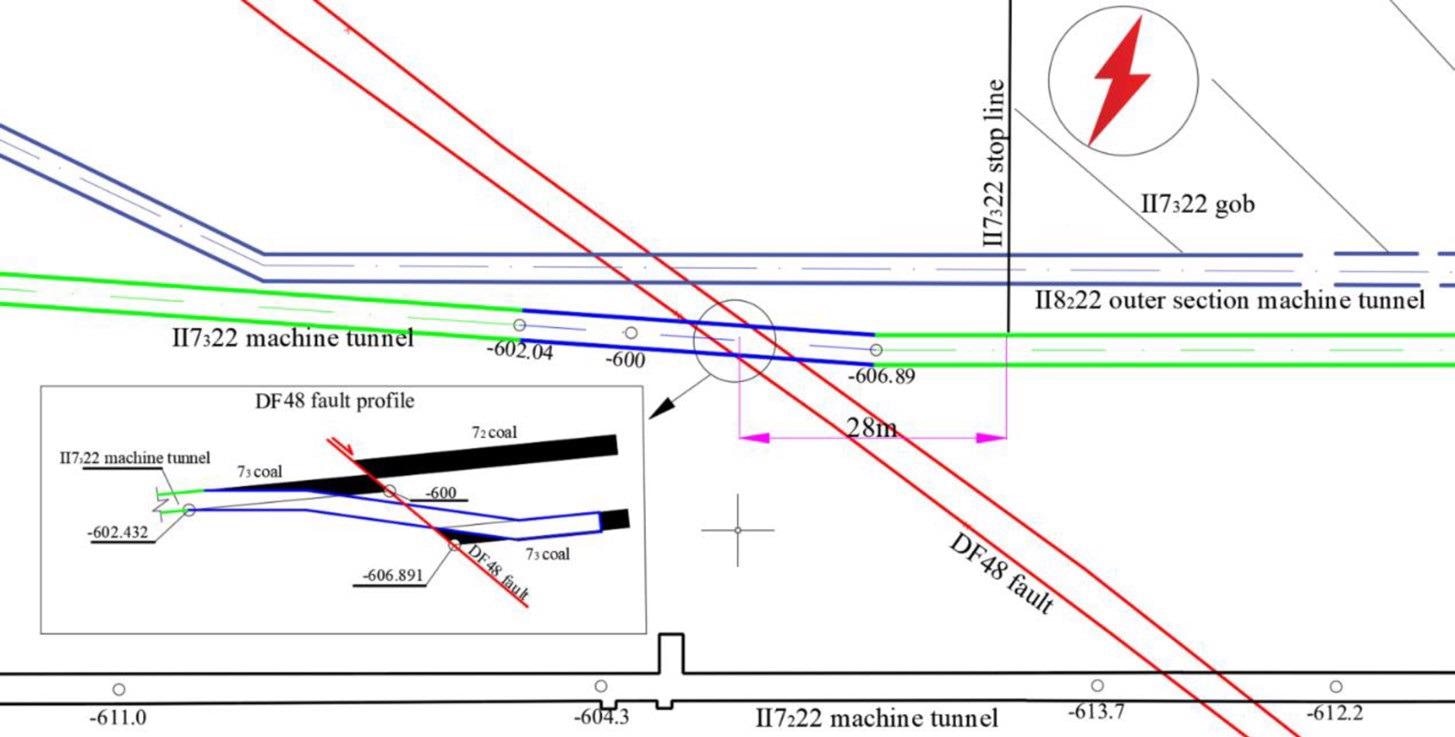
Figure 11. DF48 fault location map. Image Credit: Wang, et al., 2022
Conclusion
An experimental system was built and an explosive accident study was undertaken in this work, based on micro-mechanical examination of sandstone samples from the Renlou mine, to examine the probability of a piezoelectric effect igniting methane in the gob, with the following primary conclusions:
(1) The piezoelectric effect and compressive strength are important elements in the collecting of free charges before sandstone fracture.
(2) The rock tip holds a large number of charges at the time of sandstone fracture, generating a “point-surface” effect that causes the electron avalanche.
(3) The spark is orange-yellow and lasts for 22 milliseconds during the electron avalanche, significantly exceeding the ionization energy and ignition induction duration of methane.
(4) The root causes of the creation of electrical discharges and ignition of the gas explosion in the Renlou coal mine II7322 gob are the piezoelectric action and compressive strength of the sandstone.
Journal Reference:
Wang, Y.-N., Wang, D.-M., Xin, H.-H., Zhu, Y.-F., Hou, Z., Zhang, W., Li, M. (2021) Piezoelectric effect and ignition characteristics of coal mine gob roof collapse. ACS Omega, 6(43), pp. 28936–28945. Available Online: https://pubs.acs.org/doi/10.1021/acsomega.1c03987?ref=pdf&.
References and Further Reading
- Brodny, J., et al. (2017) M. A data warehouse as an indispensable tool to determine the effectiveness of the use of the longwall shearer. In: International Conference: Beyond Databases, Architectures and Structures; Springer. pp. 453−465 doi.org/10.1007/978-3-319-58274-0_36.
- Wang, D (2018) Thermodynamic disasters and characteristics of coal mines. Journal of China Coal Society, 43, pp. 137−142.
- Wang, D (2018) Thermodynamic disasters in coal mines. Science Press, pp. 14−37.
- Tutak, M & Brodny, J (2017) Determination of particular endogenous fires hazard zones in goaf with caving of longwall. IOP Conference Series: Earth and Environmental Science; IOP Publishing, 95(4). doi.org/10.1088/1755-1315/95/4/042026.
- Ma, D., et al. (2019) Study on the methane explosion regions induced by spontaneous combustion of coal in longwall gobs using a scaled-down experiment set-up. Fuel, 254, p. 115547. doi.org/10.1016/j.fuel.2019.05.130.
- Li, L., et al. (2020) Integrated experimentation and modeling of the formation processes underlying coal combustion-triggered methane explosions in a mined-out area. Energy, 203, p. 117855. doi.org/10.1016/j.energy.2020.117855
- Chen, X., et al. (2021) Distribution and prevention of CO in a goaf of a working face with Y type ventilation. ACS Omega, 6(3), pp. 1787−1796. doi.org/10.1021/acsomega.0c02853
- Pan, R. K., et al. (2020) Evolution patterns of coal micro-structure in environments with different temperatures and oxygen conditions. Fuel, 261, p. 116425. doi.org/10.1016/j.fuel.2019.116425.
- Xu, J. C., et al. (2002) Study on the method for determining dangerous zones of coal self-ignition in gobs in a fully mechanized top-coal caving face. Journal of University of Science and Technology of China, 32(6), pp. 672−677. Available at: https://oversea.cnki.net/kcms/detail/detail.aspx?dbcode=cjfd&dbname=cjfd2002&filename=ZKJD200206006.
- Zhou, F B (2012) Study on the coexistence of gas and coal spontaneous combustion(I): disaster mechanism. Journal of China Coal Society, 37(5), pp. 843−849. Available at: https://www.ingentaconnect.com/content/jccs/jccs/2012/00000037/00000005/art00023.
- Zhou, X (2006) Study on spontaneous combustion and explosion characteristics and prevention and control technology with high-gas and easily-spontaneous combustion coal seam in stope. Liaoning Technical University.
- Ward, C. R., et al. (2001) Identification of potential for methane ignition by rock friction in Australian coal mines. International Journal of Coal Geology, 45, pp. 91−103. doi.org/10.1016/S0166-5162(00)00025-2.
- Qu, Q. D., et al. (2006) Experimental study on gas explosion detonated by the rock friction sparks. Journal of China Coal Society, 31(4), pp. 466−469. Available at: https://www.safetylit.org/citations/index.php?fuseaction=citations.viewdetails&citationIds[]=citjournalarticle_70158_8.
- Xu, J., et al. (2007) Study on gas explosion induced by impact-friction sparks during roof collapse with fully-mechanized top-coal caving mining. International Journal of Mining Science and Technology, 36(1), pp. 12−16. Available at: https://www.osti.gov/etdeweb/biblio/21004958.
- Wang, J.-C., et al. (2007) Experimental research on gas hazard incident caused by roof collapse. Journal of Mining & Safety Engineering, pp. 12−16.
- Brady, B T & Rowell, G A (1986) Laboratory investigation of the electrodynamics of rock fracture. Nature, 321, pp. 488−492. doi.org/10.1038/321488a0.
- Kato, M., et al. (2010) Photographic evidence of luminescence during faulting in granite. Earth, Planets and Space, 62, pp. 489−493. doi.org/10.5047/eps.2010.02.004.
- Takaki, S & Ikeya, M (1998) A dark discharge model of earthquake lightning. Japanese Journal of Applied Physics, 37(9R), p. 5016. doi.org/10.1143/JJAP.37.5016.
- Widom, A., et al. (2013) Neutron production from the fracture of piezoelectric rocks. Journal of Physics G: Nuclear and Particle Physics, 40, p. 015006. doi.org/10.1088/0954-3899/40/1/015006.
- Aufort, J., et al. (2015) Effect of pores and grain size on the elastic and piezoelectric properties of quartz-based materials. American Mineralogist, 100(5–6), pp. 1165−1171. doi.org/10.2138/am-2015-5180ccby.
- Casals, B., et al. (2021) Avalanche criticality during ferroelectric/ferroelastic switching. Nature Communications, 12, p. 345. doi.org/10.1038/s41467-020-20477-6.
- Freund, F. T., et al. (2009) Air ionization at rock surfaces and pre-earthquake signals. Journal of Atmospheric and Solar-Terrestrial Physics, 71(17-18), pp. 1824−1834. doi.org/10.1016/j.jastp.2009.07.013.
- Li, M (2020) Study on the mechanical-electrical signature and ignition properties of coal mine goaf roof. China University of Mining & Technology.
- Yasui, Y (1971) A study on the luminous phenomena accompanied with earthquake (part II). Memoirs of Kakioka Magnetic Observatory, 14, pp. 67−77. Available at: https://ci.nii.ac.jp/naid/10003665028/.
- Haberland, H (1985) A model for the processes happening in a raregas cluster after ionization. Surface Science, 156, pp. 305−312. doi.org/10.1016/0039-6028(85)90588-6.
- Raizer, Y P & Braun, C (1991) Gas Discharge Physics. Applied Optics, 31, pp. 2400−2401. Available at: https://link.springer.com/book/9783642647604.
- Martelli, G., et al. (1989) Light, radiofrequency emission and ionization effects associated with rock fracture. Geophysical Journal International, 98(2), pp. 397−401. doi.org/10.1111/j.1365-246X.1989.tb03362.x.
- Lintin, D R &Wooding, E R (1959) Investigation of the ignition of a gas by an electric spark. British Journal of Applied Physics, 10, pp. 159−166. doi.org/10.1088/0508-3443/10/4/302.
- Wang, H., et al. (2020) Experimental investigation of the impact of CO, C2H6, and H2 on the explosion characteristics of CH4. ACS Omega, 5(38), pp. 24684−24692. doi.org/10.1021/acsomega.0c03280.
- Liang, Y., et al. (2019) Ignition mechanism of gas in goaf induced by the caving and friction of sandstone roof containing pyrite. Process Safety and Environmental Protection, 124, pp. 84−96. doi.org/10.1016/j.psep.2019.02.005.
- Yao, L., et al. (2018) Flash heating and local fluid pressurization lead to rapid weakening in water-saturated fault gouges. Journal of Geophysical Research: Solid Earth, 123(10), pp. 9084−9100. doi.org/10.1029/2018JB016132.